Proteins. A complicated yet intriguing molecule that is critical for the shape and function of all living organisms. While the first thing that comes to mind when thinking of proteins may be your protein shake, these molecules do much more as they are important in the structure of organisms, overseeing the rate of chemical reactions in the body, binding to form cells into tissues, and more. These vastly different functions arise through a process called protein folding, which gives proteins its stability and shape. However, how exactly do proteins work and what are some applications of proteins in your body and in biotechnology?
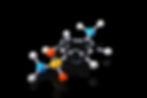
Types of Macromolecules
In biology, there are four different types of macromolecules: carbohydrates, lipids, proteins, and nucleic acids. These macromolecules are crucial for the survival and growth of an organism, for example, carbohydrates are responsible for storing energy, lipids maintain the structure of cells, nucleic acids store and pass down genetic information, and proteins have a wide variety of functions, from maintaining the structure of an organism to catalyzing chemical reactions. Some types of proteins include enzymes, which are catalysts that speed up chemical reactions by breaking up, linking, or rearranging the substrate, messenger proteins, such as hormones, which provides chemical signals to regulate physiological processes. In addition, antibodies, which you may have heard of recently due to news about vaccines and pandemics, are also proteins, and they are responsible for identifying and neutralizing pathogens. Other proteins, such as collagen or keratin, are structural proteins responsible for forming structures like the cytoskeleton, while transport proteins carry atoms and small molecules throughout the body.

This is a protein modeled by protein modeling software! Image Source
How do proteins get their function?
However, how do these proteins get their function? It all has to do with how proteins fold, and the way a protein folds has to do with the amino acid structure. Amino acids are monomers, which are molecules that can bond to make a polymer, that make up proteins. There are 20 different types of amino acids found in proteins, such as alanine, serine, glycin, and more, which have different properties that impact how the protein will fold, particularly its side chains. The side chains of an amino acid determine whether it’s hydrophilic (meaning water-loving), hydrophobic (meaning water-hating), acidic, or basic. Since the hydrophilic side chains are attracted to water, they tend to face outward while the hydrophobic chains face inward. Different amino acids have different chemical behaviors, so when hundreds or thousands of different amino acids bond together with peptide bonds to form a chain called a polypeptide, the protein folds itself into a complex 3-D shape to accommodate the amino acids.

What are the different levels of protein structures?
There are four different levels of protein structures: primary, secondary, tertiary, and quaternary. The primary structure is the order of the amino acids in the polypeptide chain, which is determined by the DNA encoding for the protein. However, the translation of the amino acids is prone to mistakes, and one single mutation can drastically change the sequence of the amino acids, and this in turn, changes the structure of the protein, which determines the function, thus changing the entire function of the protein. After the primary structure of proteins, comes the secondary structure. The secondary structure in proteins are local folded structures in polypeptides formed by hydrogen bonding between amino acids, and these often include ɑ helices that look like twisted rods and β pleated sheets that have zig-zag patterns. In addition, another protein structure is the tertiary structure, which is the overall 3-D structure of the protein created by arranging secondary structures into a final 3-D shape through bonding, including hydrogen bonds and disulfide bonds. To summarize, the order of the amino acids in the primary structure determines where ɑ helices and β pleated sheets exist, and this secondary structure folds into a 3-D shape that becomes the overall structure of a single protein. However, while many proteins can act as a single polypeptide chain, sometimes these polypeptide chains can bond together to create a quaternary structure, for example, hemoglobin and potassium channels, and these polypeptide chains are held together with hydrogen bonds or other bonds. With the different length and sequences of amino acids, there are many possibilities for protein structures, with some proteins creating hollow tubes, snakes, and pockets.

This graphic shows how denaturation turns a folded protein into an unfolded protein! Image Source
What is protein denaturation?
Yet, the correct folding of the protein is important, because the structure of the protein determines its function, so when a change in certain conditions, such as a change in pH, temperature, or environment occurs, this can break the bonds that create the secondary and tertiary structure of the protein in a process called denaturation. Denaturation often causes a loss of function, such as the loss of the ability to act as a hormone or enzyme. A common example of denaturation is in boiled eggs: The egg whites turn solid and white when cooked because the temperature breaks the bonds holding the protein together, which causes the hydrophobic amino acid sequences to be exposed to water. This in turn causes the hydrophobic amino acids to stick together into a coagulated state, forming the solid egg whites. However, while we know that a cooked egg can’t turn back into a raw egg, there are some proteins that can return to its normal function after being denatured when the conditions return to normal. For example, when milk is warmed, the proteins are slightly denatured but don’t reach coagulation, which gives it stability when denatured, so when the milk is cooled, the proteins can renature and revert back to its original form. However, how does this impact the human body? Denaturation can actually have serious impacts on the human body, as the proteins in your body can actually denature in high temperatures. To illustrate, heatstroke, which is caused by overheating and often regarded as the most severe form of heat injury, becomes dangerous because the increased temperatures can cause the denature proteins and destabilize molecules in your body, causing cardiovascular collapse, multiorgan failure, and death. This is also why fevers above 106° F are considered dangerous, since temperatures above 106° F could potentially denature the proteins in your body.

This graphic shows what happens with misfolded proteins! Image Source
What causes protein misfolding and improper protein function?
In addition to protein denaturation, there are other issues that can occur because of improper protein function. These arise from problems with transcription and translation, which is the central dogma of biology. This dogma states the nucleus of a cell contains the DNA needed to make a protein, and this DNA is transcribed by messenger RNA and carried to the ribosomes, where RNA is translated into a protein. However, this process is not completely accurate, and in fact, mutations often arise due to improper transcription or translation. The human genome contains approximately 3 billion base pairs, so it’s not hard to imagine why an error might arise when copying billions of base pairs. When this happens, the amino acid may stay the same or turn into a different amino acid, and in the case that the mutation causes a change in the amino acid, the protein may not be able to fold properly. A misfolded protein can cause the protein to be unable to work, or even create a “sticky, clumpy mess” inside the cell. One disease that is caused by protein misfolding due to mutations is cystic fibrosis, which is a disease that affects the cells that produce mucus, sweat, and digestive juices. In cystic fibrosis, a mutation in the CFTR gene causes the protein to misfold, and this causes a buildup of intracellular chloride ions. Cystic fibrosis, and other diseases such as Tay-Sachs disease or Marfan Syndrome, are caused by a single mutation on the DNA sequence encoding for a protein. However, even if the protein has been transcribed properly, mistakes can still occur when the chain of amino acids are being produced in the ribosome, which are estimated to happen in 1 out of every 7 proteins produced! In addition, proteins can also make mistakes during folding, as proteins do not fold correctly every single time. When a protein misfolds, it can result in a “loss-of-function” protein, which is illustrated by Kerry Geiler as “Imagine that a properly folded protein is perfectly shaped to bind a toxin and break it into less toxic byproducts. Without enough of the properly folded protein available, the toxin will build up to damaging levels.” Misfolded proteins can also result in clumps that stick together in the core of the protein because of the carbon-rich amino acids on their surfaces that tend to aggregate, and this can cause diseases such as Alzheimer’s, Huntington’s, ALS, and more.
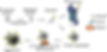
This graphic shows how the misfolded proteins get destroyed or aggregate! Image Source
How does the body prevent misfolded proteins?
However, your body has many mechanisms to prevent misfolded proteins. Just like quality control at manufacturing facilities, cells have their own quality control to prevent faulty proteins from existing. One way cells achieve this is through a special protein called chaperones, which serve to assist in protein building and ensure that only properly folded proteins can continue to the secretory pathway. These proteins can shelter proteins that are in the process of folding to prevent other molecules from interfering with the folding process. In fact, since heat can destabilize proteins, these chaperone proteins are often called “heat shock” proteins because they are made in large amounts when the cell experiences heat, since proteins tend to misfold when destabilized. There are two types of chaperone proteins: molecular chaperones, which prevent unfolded or partially folded proteins from being degraded by stabilizing them, and chaperonins, which directly assist in protein folding. But in addition to chaperones, cells also have proteasomes, which are protein complexes that are responsible for degrading proteins and peptides that have been marked by ubiquitin through proteolysis, which is a process that breaks peptide bonds. All of these mechanisms are used by the cell to prevent misfolded proteins from existing, but sometimes these mechanisms are not enough, and the misfolded protein can cause disease.
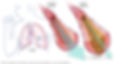
This graphic shows how the mucus affects the lungs in cystic fibrosis patients! Image Source
How have proteins shaped science?
While proteins are important in the human body, they have also shaped science as proteins have played a crucial role in drug discovery and deepened our understanding in cellular processes and pathophysiology. For example, since Max Perutz and John Kendrew discovered the structure of globular proteins in 1962, scientists have discovered nearly 80,000 protein structures, and because of our understanding of proteins and biology, the survival rates of diseases such as cystic fibrosis have increased dramatically throughout the years, as the median survival rate of cystic fibrosis is now over 40 years. While so far there is no definitive cure for cystic fibrosis, the Food and Drugs Administration (FDA) recently approved a treatment called Trikafta, which is a combination of three drugs that targets the faulty CFTR protein. With these advancements in modern medicine propelled by our understanding of proteins, scientists may one day discover more drugs and treatments available for diseases that are currently incurable, such as Huntington’s Disease or Alzheimer’s Disease, both of which are caused by misfolded proteins. However, many discoveries would not be possible without the help of protein modeling technology, which can model the 3-D structure of a protein with data predicted from in-silico techniques using computational technology. Computer software such as PyMOL, Jmol, Rosetta, and more, allow laboratories and educational institutions to visualize the structure of proteins, and protein folding games such as Foldit from the University of Washington are even making protein modeling more accessible to the general public! In fact, Foldit is a free puzzle game that allows players without previous experience in biochemistry to solve puzzles for science, and I personally think it’s a fun way to learn about proteins especially with the little bubbles that provide information about proteins and bonding!
In conclusion, proteins are a crucial part of life, in our body as well as in science, as the human body is essentially made of proteins. With over 20,000 to 100,000 different proteins in a single human cell but only 20 different amino acids, the function of the protein is greatly dependent on the structure of the protein, which is determined by the sequence of amino acids. However, this process is far from perfect, as mutations and mistakes during folding can cause severe diseases including cystic fibrosis, Tay-Sachs Disease, Marfan Syndrome, Alzheimer’s, and Huntington’s. Nevertheless, the body has mechanisms that prevent misfolded proteins, such as chaperones and proteasomes, and our understanding of proteins have potentially allowed scientists to one day cure the diseases caused by protein misfolding faced by millions of people worldwide.
Bibliography
Geiler, K. (2014, January 09). Protein Folding: The Good, the Bad, and the Ugly. Retrieved December 04, 2020, from http://sitn.hms.harvard.edu/flash/2010/issue65/
Bailey, R. (2019, May 4). Learn About the 4 Different Types of Protein Structure. Retrieved December 04, 2020, from https://www.thoughtco.com/protein-structure-373563
Protein structure: Primary, secondary, tertiary & quaternary (article). (n.d.). Retrieved December 04, 2020, from https://www.khanacademy.org/science/biology/macromolecules/proteins-and-amino-acids/a/orders-of-protein-structure
Protein denaturation. (n.d.). Retrieved December 04, 2020, from https://www.britannica.com/science/protein/Protein-denaturation
Quaternary Structure. (n.d.). Retrieved December 04, 2020, from https://cbm.msoe.edu/teachingResources/proteinStructure/quaternary.html
Helman, R. S., M.D. (2020, September 08). Heat Stroke. Retrieved December 04, 2020, from https://emedicine.medscape.com/article/166320-overview
What is the 'Central Dogma'? (2016, January 25). Retrieved December 04, 2020, from https://www.yourgenome.org/facts/what-is-the-central-dogma
Reynaud, E. (2010) Protein Misfolding and Degenerative Diseases. Nature Education 3(9):28
PDB101: Molecule of the Month: Chaperones. (n.d.). Retrieved December 04, 2020, from https://pdb101.rcsb.org/motm/32
Stony Brook University. (2012, November 22). Protein folding: Look back on scientific advances made as result of 50-year old puzzle. ScienceDaily. Retrieved December 3, 2020 from www.sciencedaily.com/releases/2012/11/121122152910.htm
Office of the Commissioner (2019, October 21). FDA approves new breakthrough therapy for cystic fibrosis. Retrieved December 04, 2020, from https://www.fda.gov/news-events/press-announcements/fda-approves-new-breakthrough-therapy-cystic-fibrosis
What are proteins and what do they do?: MedlinePlus Genetics. (2020, September 18). Retrieved December 04, 2020, from https://medlineplus.gov/genetics/understanding/howgeneswork/protein/
Keller, B. (2016, April 01). Making Sense of Medicine: Denature and/or die. Retrieved December 04, 2020, from https://www.newburyportnews.com/news/lifestyles/making-sense-of-medicine-denature-and-or-die/article_f62775fb-b4e4-5bab-abb6-835ace8603ce.html
Lodish H, Berk A, Zipursky SL, et al. Molecular Cell Biology. 4th edition. New York: W. H. Freeman; 2000. Section 3.2, Folding, Modification, and Degradation of Proteins. Available from: https://www.ncbi.nlm.nih.gov/books/NBK21750/
How the proteasome is degraded, Daniela Hoeller, Ivan Dikic, Proceedings of the National Academy of Sciences Nov 2016, 113 (47) 13266-13268; DOI: 10.1073/pnas.1616535113
Fraser-Pitt, D., & O'Neil, D. (2015). Cystic fibrosis - a multiorgan protein misfolding disease. Future science OA, 1(2), FSO57. https://doi.org/10.4155/fso.15.57