In 2010, Craig Venter and his team in La Jolla, California synthesized the smallest bacterial genome with just 473 genes. This was a huge advancement in the field of synthetic biology, where scientists create and redesign biological systems that don’t exist naturally, such as creating life through a computer software. By transplanting the Mycoplasma mycoides bacteria, a parasite that affects cattle and other ruminants, into the Mycoplasma capricolum bacteria, Craig Venter and his team were able to synthesize a cell that is controlled by the donor genome. As discoveries in synthetic biology continue progressing, scientists may one day be able to change medicine, technology, environmental science, and more. In fact, according to Jay Keasling, a professor at the University of California, Berkeley, “In medicine, the synthetic biology community is pushing the boundaries by designing microbes that will seek and destroy tumors in the body before self-destructing. Synthetic biology also provides us a way to clean up our environment. We can build organisms to consume toxic chemicals in water or soil that would not otherwise decompose, for example.”

This graphic shows how Sanger Sequencing works! Image Source
In the 1950s, the race to discover the structure of DNA was on, with James Watson, Francis Crick, Rosalind Franklin, and Maurice Wilkins eventually discovering the structure of DNA using the crucial photograph, “Photo 51,” taken by Rosalind Franklin and a graduate student, Raymond Gosling. At the time, the technology available was not sufficient to sequence the DNA, which is the process of determining the order of the base pairs. But by the 1970s, scientists had developed methods of sequencing DNA, including a popular method used for much of the Human Genome Project called Sanger Sequencing. Sanger Sequencing was developed by Fredrick Sanger, who was a British biochemist that was the first to sequence the genome of the bacteriophage Phi X 174. In Sanger Sequencing, primers, DNA polymerase, nucleotides, dideoxynucleotides are added in a test tube with the DNA sequence that has been amplified. This mixture is heated up to denature the strands of DNA, which will allow primers to bind to the individual strands. Then, when a primer has bound to the strand of DNA, nucleotides in the mixture will continuously bind to the strand of DNA until a dideoxynucleotide is added to the chain, which will stop the chain. This is because the dideoxynucleotide does not have a hydroxyl group on the 3’ carbon of the sugar ring, while a regular nucleotide has the 3’ hydroxyl group, which allows it to connect to other nucleotides and continue the sequence. These dideoxynucleotides have dyes attached to nitrogenous bases, and the different dyes correspond with each of the nucleotide bases, so for example, adenine is typically green, thymine is red, cytosine is blue, and guanine is yellow. The entire process is repeated multiple times for the same DNA strand, so after a certain amount of cycles, all of the nucleotides in the sequence will have a dideoxynucleotide attached to it. This means that the test tube will contain chains of different lengths that end in a specific color to mark the final nucleotide, and the chains will be run through capillary gel electrophoresis to separate the sequences by length, which will determine the order of the nucleotides.
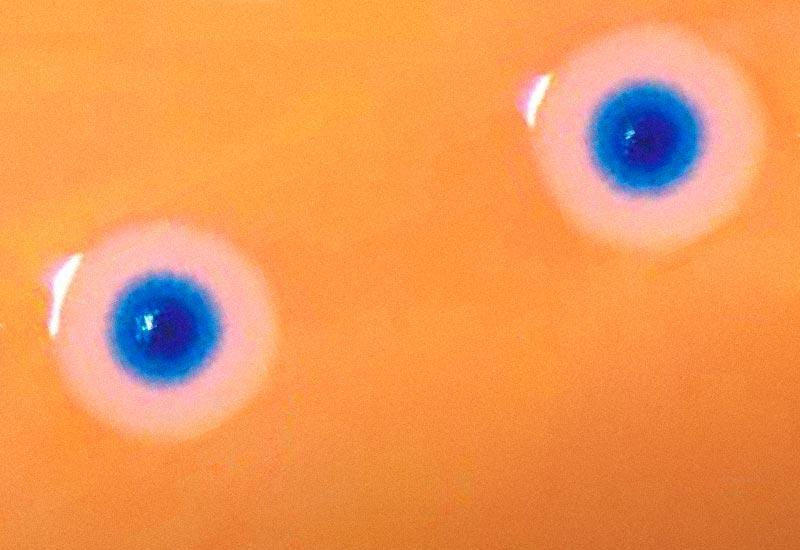
These are the synthetic cells created by Craig Venter and his team! Image Source
By 1995, Craig Venter and his team used the sequencing technology to sequence the genome of a self-replicating bacteria, Haemophilus influenzae, and since then, sequencing technology has simultaneously become cheaper and easier with the invention of next-generation sequencing. However, one question still persists: Will scientists be able to synthesize an organism? For 15 years, Craig Venter and his team worked on developing a strategy to synthesize an organism, and in 2008, they built the Mycoplasma genitalium bacteria, which is the smallest genome of an organism capable of being grown in a laboratory, by constructing virus-sized cassettes of DNA to produce a large DNA molecule. By creating a genome from a DNA sequence on the computer, this was the first synthetic genome ever created. However, this time, Venter’s team wanted to create a cell that could be controlled by the synthetic genome, and they decided to use the Mycoplasma mycoides as the donor species, and Mycoplasma capricolum as the recipient species because of the faster growth rate. The sequences used to synthesize the bacteria were based on previous genome sequences, and the accuracy of the sequence was critical to the success of the experiment. The experiment was done in four stages, and during the process, the scientists first recombined the gene cassettes, which were chemically synthesized with three chemicals by Blue Heron Biotech, and vectors in yeast, which were then transferred to Escherichia coli. Then, the intact synthetic M. mycoides was extracted and transferred to the recipient cells, M. capricolum. Through electrophoresis, the scientists found that there were a couple differences between the synthetic cells and the wild-type control cells, but no lethal mutations were found. To identify the synthetic cell from the wild-type cells, the scientists added watermark sequences that did not code for proteins but gave the cell several distinctive markers. In total, the synthetic genome started as a genome sequence in the computer that was then grown as a yeast centromeric plasmid by stitching together pieces of the genome that were manufactured synthetically, and this genome was inserted into a recipient cell. The resulting cell was controlled by the donor genome, as the proteins made were those of the donor cell, and it could self-replicate to make more copies of the cell.
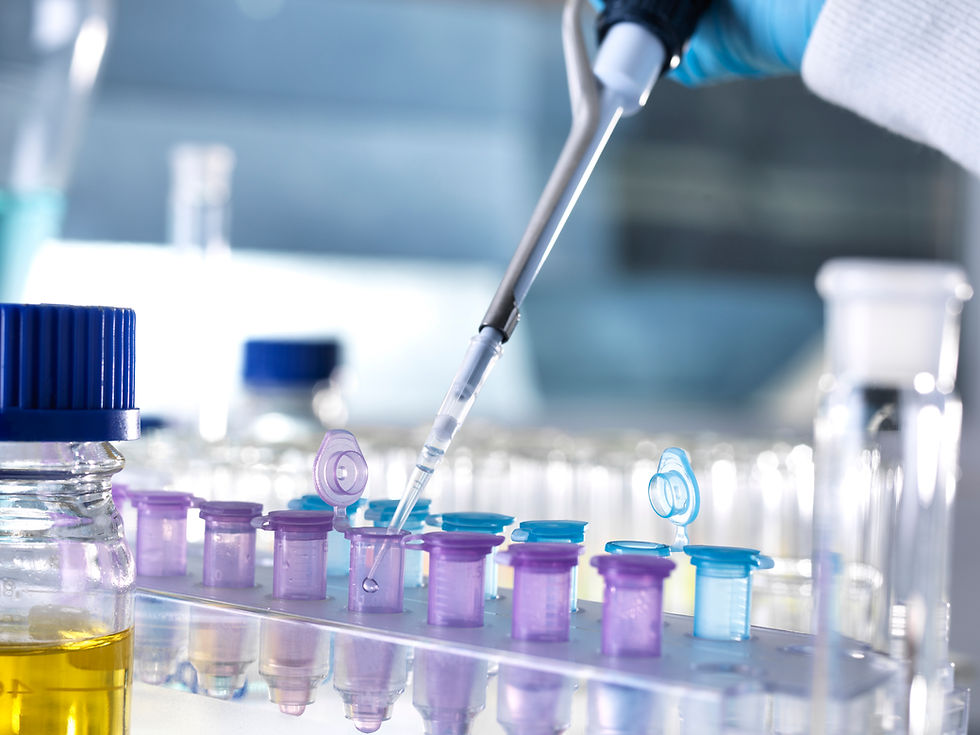
According to Craig Venter, while they have created the first synthetic cell, the scientists have not created life from scratch because even though the genome was synthesized from a sequence designed on a computer, the bacterial genome was created by bacteria and yeast, which was then finally booted up in a recipient cell. This process cost in total $40 million and 15 years, which leaves many people wondering, if we can just alter existing organisms through genetic engineering, why do we need synthetic biology? One of the main pursuits in synthetic biology is the development of biofuels, as with our current rate of fossil fuels consumption, it will take us 53 years before we run out of fossil fuels, which are an nonrenewable resource. However, biofuels, which come from ethanol produced by corn, soybeans, and other biomass, are considered renewable, and this could help us produce a safer and cleaner alternative to fossil fuels. With the use of synthetic biology, microorganisms could help produce energy, as it was shown by Craig Venter’s project that the M. capricolum could be controlled by the donor cell, so bacteria and other microorganisms could be engineered to efficiently produce biofuels by designing bacterial genomes optimized for producing biofuels and inserting them into bacterial cells. Similarly, insulin is produced by inserting the insulin gene into the bacterial plasmid, but this is done in the plasmid rather than with the entire bacterial genome. For insulin, the human insulin gene is added to a bacterial plasmid, which is inserted back into the bacteria, which is then grown to produce the insulin.
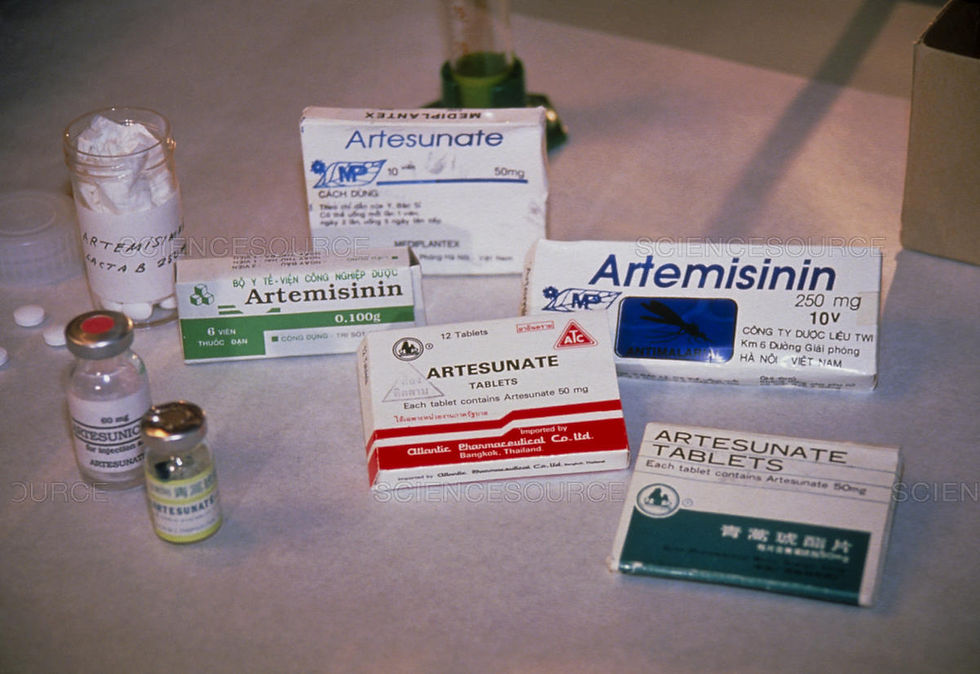
These are the antimalarial drugs, Artemisinin! Image Source
Likewise, another use for synthetic biology is in medicine. In fact, in 2003, Jay Keasling and his team at UC Berkeley announced that they had produced the precursor to the antimalarial drug, artemisinin. This was done by implanting the gene for the metabolic pathways into E. coli, which was then engineered into yeast. With this crucial development, the antimalarial artemisinin could become cheaper and more accessible as more than 435,000 deaths from malaria according to the World Health Organization (WHO). In addition, synthetic biology has also been used in the pharmaceutical industry by revolutionizing drug delivery methods. For example, CHAIN Biotechnology has developed a new living drug using Clostridium bacteria and engineering it to carry therapeutic metabolites, peptides, and enzymes. This drug can be taken orally, and once taken, the bacteria will germinate in the gut and secrete the therapeutics. According to an article published in Medical Principles and Practice, “[metabolic engineering in synthetic biology] can also be applied to microorganisms to produce chemicals more efficiently than by genetic engineering.”
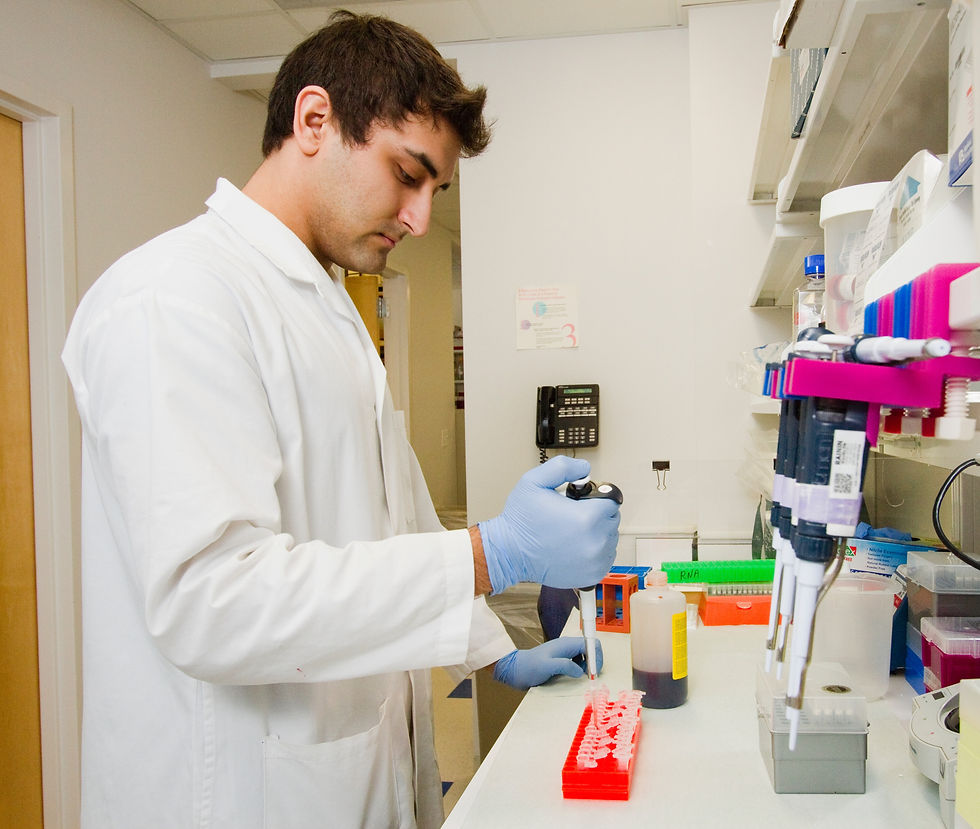
However, there’s more applications to synthetic biology than in just healthcare and biofuels. In agriculture, scientists at MIT transferred genes from bacteria into cereal crops so the crops will be able to fix their own nitrogen from the air, reducing the need for chemical fertilizers. Other uses for synthetic biology in agriculture include improving the nutritional content of food, synthesizing metabolic pathways of plants to reduce the amount of carbon emissions in the atmosphere, and improving agriculture production. Even the cosmetics industry has been impacted by synthetic biology, as biosynthesis is now changing the way companies are producing makeup products. Squalene, which is typically used for moisturiser and antioxidants, has been produced by biotechnology company Amyris through biosynthesis. Many companies are using synthetic biology to produce sustainable products, which reduces the use of petroleum products and animal-based products. The fashion industry is another industry that has been using synthetic biology to create more sustainable products. To illustrate, jeans are typically dyed with a variety of chemicals and petroleum products, but Tinctorium Bio has created a dye similar to indigo by producing the precursor to indigo, called indican, using E. coli. An enzyme is added to the indican to make leucoindigo, which is the same dye that is typically used to dye jeans.
Synthetic biology has huge applications, from revolutionizing the delivery of drugs to replacing the use of petroleum in products that we use in our daily lives. In addition, the discovery by Craig Venter and his team may lead to further findings, as scientists are now able to design genomes and synthesize bacteria. With these discoveries, we may be able to create sustainable alternatives to petroleum products and improve global health and nutrition. As the field of synthetic biology is rapidly progressing, who knows what we will be able to do in the future?
Bibliography
Gibson, D. G., Glass, J. I., Lartigue, C., Noskov, V. N., Chuang, R., Algire, M. A., . . . Venter, J. C. (2010). Creation of a Bacterial Cell Controlled by a Chemically Synthesized Genome. Science, 329(5987), 52-56. doi:10.1126/science.1190719
Keasling, J. (2013, February 28). Why Synthetic Biology Is the Field of the Future. Retrieved November 11, 2020, from https://www.pbs.org/wgbh/nova/article/why-synthetic-biology-is-the-field-of-the-future/
Scientist: 'We didn't create life from scratch'. (2010, May 21). Retrieved November 11, 2020, from https://www.cnn.com/2010/HEALTH/05/21/venter.qa/index.html
When will fossil fuels run out? (n.d.). Retrieved November 11, 2020, from https://www.ecotricity.co.uk/our-green-energy/energy-independence/the-end-of-fossil-fuels
How did they make insulin from recombinant DNA? (n.d.). Retrieved November 11, 2020, from https://www.nlm.nih.gov/exhibition/fromdnatobeer/exhibition-interactive/recombinant-DNA/recombinant-dna-technology-alternative.html
Synthetic Biology Explained. (n.d.). Retrieved November 11, 2020, from https://archive.bio.org/articles/synthetic-biology-explained
Sawyer, E. (2011, June 23). Artemisinin: A Synthetic Biology Success Story. Retrieved November 11, 2020, from https://www.nature.com/scitable/blog/bio2.0/artemisinin_a_synthetic_biology_success/
Raper, V., Ph.D. (2019, December 10). Synthetic Biology Seizes New Ground in Healthcare. Retrieved November 11, 2020, from https://www.genengnews.com/insights/synthetic-biology-seizes-new-ground-in-healthcare/
Miller, L. (2020, January 10). Making real a biotechnology dream: Nitrogen-fixing cereal crops. Retrieved November 12, 2020, from https://news.mit.edu/2020/making-real-biotechnology-dream-nitrogen-fixing-cereal-crops-0110
Roell, M., & Zurbriggen, M. D. (2020). The impact of synthetic biology for future agriculture and nutrition. Current Opinion in Biotechnology, 61, 102-109. doi:10.1016/j.copbio.2019.10.004
Cumbers, J. (2019, September 10). Synthetic Biology Is Disrupting Personal Care, Making It More Sustainable - And More Personal. Retrieved November 12, 2020, from https://www.forbes.com/sites/johncumbers/2019/09/10/synthetic-biology-is-disrupting-personal-care-making-it-more-sustainable-and-personal/?sh=20977b3047c5
Gallegos, J. E. (2020, February 18). Synthetic biology is shaking up these 5 industries-Some of them might surprise you. Retrieved November 12, 2020, from https://synbiobeta.com/synthetic-biology-is-shaking-up-these-5-industries-some-of-them-might-surprise-you/
Callaway, E. (2016, March 24). 'Minimal' cell raises stakes in race to harness synthetic life. Retrieved November 12, 2020, from https://www.nature.com/news/minimal-cell-raises-stakes-in-race-to-harness-synthetic-life-1.19633
Callaway, E. (2010, May 20). Immaculate creation: Birth of the first synthetic cell. Retrieved November 12, 2020, from https://www.newscientist.com/article/dn18942-immaculate-creation-birth-of-the-first-synthetic-cell/
Sanger Sequencing Steps: DNA Sequencing. (n.d.). Retrieved November 12, 2020, from https://www.sigmaaldrich.com/technical-documents/articles/biology/sanger-sequencing.html
Sanger F, Air GM, Barrell BG, Brown NL, Coulson AR, Fiddes CA, Hutchison CA, Slocombe PM, Smith M. Nucleotide sequence of bacteriophage phi X174 DNA. Nature. 1977 Feb 24;265(5596):687-95. doi: 10.1038/265687a0. PMID: 870828.
Heather, J. M., & Chain, B. (2016). The sequence of sequencers: The history of sequencing DNA. Genomics, 107(1), 1–8. https://doi.org/10.1016/j.ygeno.2015.11.003
Comments